Welcome to Fong’s Lab!
Meet the gang!

From left to right: William Leopold, Ryan Borgman & Morgan Nieman
My name is William Leopold – I’m a music and biology major, and I’m from the best state in this country (New Jersey). In the lab, you can find me carrying too much glassware at once, gently carrying frogs to that big pond in the sky, staring at tadpoles until they grow front legs, and begging Dr. Fong to stop gatekeeping his best fishing spots. Outside of lab, I spend most of my time practicing piano, or honing my breaking and entering skills when the rooms with good pianos in Schmucker hall are locked. Once I’ve practiced enough that I feel I have to get a life, I’ve had fun this summer with volleyball games, trips to Dorney park, and trying to catch fish in the sweltering heat.
Hi, my name is Ryan Borgman, I’m from Scituate, Massachusetts and I am a rising junior majoring in BMB. So far, I’ve really enjoyed my time here during the X-Sig program. When not in the lab I like to spend my time going to the gym, playing basketball and watching the Boston Celtics win their 18th NBA championship. I’ve also explored the surrounding areas with day trips to DC and Hershey Park and an upcoming trip to see world renowned Hootie and Blowfish Perform in Hershey Stadium. I chose this lab because it’s very hands on, I love working with the frogs and going out into the stream to collect various organisms like snails and clams. In the lab you can find me, graciously allowing frogs to visit with their ancestors and asking Dr. Fong “How bout those Celtics?”
Hi everyone! My name is Morgan Nieman, and I’m a rising sophomore and a biochemistry and molecular biology major. I’m from New Jersey and this is my first summer participating in X-Sig, but I have been working in the lab since last semester. In the lab you’ll find me cleaning 24/7, triple checking that we have done everything for the day, and guiding frogs to their hoppy place (personally I like to think they’re going on vacation). Outside of the lab, you’ll find me working out, going on battlefield walks, cooking, and working in town.
And most importantly — you’ll get to meet our frogs!
Research Background:
Aquatic pollution and global warming are rapidly growing environmental issues in the U.S.. Runoff from sewage treatment plants has become rampant as more antidepressants and environmental chemicals are entering natural waterways through human urination and defecation. This increase of antidepressants in the ecosystem has been observed to slow the development of aquatic organisms. Global warming also poses a threat to aquatic organisms as their environmental temperature rises drastically, they are significantly impacted developmentally. This is an incredibly pressing concern, as global warming is continuing to raise the environmental temperature. Previous research on tadpoles predicts that environmental chemicals such as antidepressants and gold nanoparticles affect the body size at metamorphosis and may cause delays in development. Furthermore, in tadpoles, increased environmental temperature has been shown to increase the rate of metamorphosis.
In all, frogs are in global decline due to pollution, climate change, pathogens, etc. Our research focuses on how climate change and pollution by gold nanoparticles (AuNPs) affect life cycle of wood frogs, specifically on their life span and body mass. In addition, how climate change affects the uptake of gold nanoparticles by tadpoles and frogs.
Experimental Set-Up:
There are seven experimental groups per temperature treatment. Our temperature treatments are 15°C, 20°C, and 25°C.
The experiment groups include:
- Citrate (control) for half larval life [28 days].
- AuNPs for half larval life [28 days].
- Citrate (control) for full larval life [at metamorphosis].
- AuNPs for full larval life [at metamorphosis].
- Life cycle exposure to citrate.
- Life cycle exposure to water.
- Life cycle exposure to AuNPs.
So, there are a total of 21 groups for this experiment. (Which leaves us with a lot of containers!)
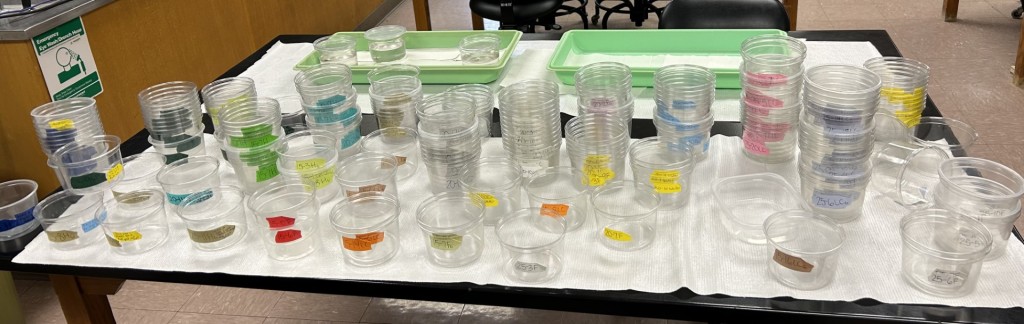
Ribbit-ing Experimental Design:
In order to expose our growing tadpoles to controlled temperatures and gold nanoparticles (or a lack of), we devised a complex and sophisticated experimental design that allows for both control and convenience. Essentially, we have several plastic containers filled with water, or citrate, or gold nanoparticles dissolved in citrate. The tadpoles call these small tupperwares their home, and we change their water / citrate / gold solution for a fresh batch once a week. As I said, high tech. Our tadpoles sit eagerly in these plastic containers until it’s finally time for their special day. When we do our daily round and see that one of them has sprouted front legs, we promote the soon-to-be froglet to a higher class of living – the same container, but with much less solution and now sporting two seashells for the froglet to stand on.
Look how much he loves his seashell! We give them many comforts in their final moments. Once the last traces of the tail are finally gone and the froglet has completed its metamorphosis, it is ready for its one true purpose – euthanasia. While we are all sad to see them go, we record their mass and then tearfully wave them across the rainbow bridge. Once the frog is fully submerged in the tube of ethanol, it is ready to be sent to Dr. Thompson’s lab, where talented chemists will analyze just how much gold nanoparticles that frog has taken up over the course of its short life. Since the gold particles are contained in a citrate solution, a citrate control group without nanoparticles is also analyzed. Our three temperature groups allow us to assess how temperature affects the uptake of gold nanoparticles, as well as how temperature affects growth and development of the frog, which we measure through time to metamorphose as well as their mass taken just before the coup de grâce. Towards the beginning of the summer, a number of tadpoles at roughly the halfway point in development were also weighed and prepared for analysis, as a means of making the same assessments as the frogs, but at a much earlier developmental stage. By using the numbered system of Gosner stages, we were able to numerically categorize just how far along each tadpole was in development at the time of death, providing us with additional insight into how both temperature and gold exposure can affect the speed at which they development, as well as how their size is affected at any stage.
Our Results (so far…):
In our experiment we’ve discovered that the temperature has a significant impact on the size and time to metamorphose of these wood frogs. The frogs in the colder water (15 degrees) metamorphose much slower than the other warmer temperatures, the warmer temperatures grew four limbs before the 15 degrees. We found that although the metamorphose slower the size of the 15-degree frogs were much larger than the warmer temperatures. The warmest temperature (25 degrees) have metamorphosed faster than the other two temperatures but they are the smallest in size. The 20 degrees are somewhere in the middle. They metamorphosed faster than the 15 but slower than the 25 and were smaller than the 15’s and larger than the 25’s on average. These results tell us that the warmer temperatures result in faster metamorphosis in the frogs and this faster metamorphosis means that the frogs are much smaller due to less growing time before they begin to metamorphose.
This can present problems in our world today with rising temperature due to global warming. The rising temperature means the frogs out in nature will start to metamorphose faster, meaning they will be full-grown frogs and leave the safety of their vernal pools much earlier and much smaller than they should be. Due to their smaller size, they will be subject to predation from a multitude of organisms. This can create a significant decrease in the frog population and throw the entire ecosystem into a frenzy.
We also looked at how pollution in our lakes and ponds may affect the metamorphosis of the wood frogs. We treated a group of tadpoles with a gold solution to look at how the gold in the water affects the absorption rate in the frogs. Gold represents pollutants that may be present in our bodies of water. After the frogs metamorphose, we process and send them to Professor Thompson’s lab, who then can tell us how much of the gold nanoparticles were absorbed by each frog. We also wanted to look at how gold impacts the rate of metamorphosis in frogs. So far, the data is looking like the frogs in the gold solution are metamorphosing faster, however this is after just briefly looking at the data, not all data points are in yet and no significant tests have been run yet.

That’s it for now. We hope you enjoyed catching up with Fong’s lab!